- Utility Menu


Harvard Natural Sciences Lecture Demonstrations
1 Oxford St Cambridge MA 02138 Science Center B-08A (617) 495-5824
- Key to Catalog
enter search criteria into the search box
Α, β, γ penetration and shielding, what it shows.
The interactions of the various radiations with matter are unique and determine their penetrability through matter and, consequently, the type and amount of shielding needed for radiation protection. Being electrically neutral, the interaction of gamma rays with matter is a statistical process and depends on the nature of the absorber as well as the energy of the gamma. There is always a finite probability for a gamma to penetrate a given thickness of absorbing material and so, unlike the charged particulate radiations which have a maximum range in the absorber where all are stopped regardless of source strength, some gammas will always get through and, given a strong enough source, a lot may get through.
How it Works
This demo is usually presented in conjunction with the Sources and Detection demo; details about the radionuclides and detectors used here can also be found in that writeup .
(1) Alpha particles interact with matter primarily through Coulomb forces between their positive charge and the negative charge of the atomic electrons within the absorber. The range of alphas of a given energy is a fairly unique quantity in a specific absorber material. [1] For a given energy, alpha particles are much slower than beta particles, giving rise to greater impulses. Additionally, its double charge (+2 e ) makes an alpha particle have a very high rate of energy loss in matter, thus making it heavily ionizing radiation. Consequently, the penetration depth of alpha particles is very small compared to the other radiations. For low density materials, the range [2] of 5.5 MeV alphas (from Am-241) is between 4.5 to 5 mg/cm 2 ; higher density materials give a range between 5 and 12 mg/cm 2 . The table below gives some specific values.
The thickness of a single sheet of paper (0.0035") is enough to stop all the alphas. [3]
(2) Beta particles also interact through Coulomb forces with the atomic electrons. Betas have much higher speeds due to their smaller mass, and smaller impulses are involved in collisions. Their penetration into matter is thus considerably greater than alphas, but because of the nature of the Coulomb force interactions, betas too are stopped by very little matter (compared to gammas). Because their masses are identical to the scattering electrons, large deviations in the beta particle path are possible, and even thin absorbers will attenuate betas by virtue of the fact that they readily get scattered out of the direct beam. Another difference that complicates the comparison is that, unlike mono-energetic alphas, beta particles come in a continuous spectrum of energies, with the average energy being about 1/3 the maximum. The low energy betas are rapidly attenuated.
A useful rule-of-thumb for the maximum range of electrons is that the range (in gm/cm 2 ) is half the maximum energy (in Mev). [4] This is of course complicated by the density: electron ranges tend to be about 2 mm per MeV in low-density materials, and about 1 mm per MeV in medium density absorbers. For our Sr/Y-90 source (maximum beta energy = 2.27 MeV, average energy = 1.13 MeV), more precise beta ranges are tabulated below:
C-14 gives off betas with a maximum energy of 0.156 MeV and average energy of 0.049 MeV. The maximum range of C-14 betas is only 0.25 mm (0.01") in plastic. We have both sources and it's nice to contrast them. A Harvard ID card is about 0.8 mm thick and stops all C-14 betas. Not so when you switch over to the Sr-90 source. A 3/8" (9.6 mm) thick piece of plastic is required to stop all the Sr-90 betas.
(3) Gamma ray interactions with matter are entirely different from that of charged particles. The lack of charge eliminates Coulomb interactions and allows gamma rays to be much more penetrating. The interactions that do occur are by way of the photoelectric effect, Compton scattering, and pair production. The probability for any of these happening is specified by a cross section, and the linear attenuation coefficients for gamma rays are defined by these cross sections.
Since linear attenuation coefficients vary with the density of the absorber, even for the same absorber material, the mass attenuation coefficient μ/ρ (linear attenuation coefficient μ in 1/cm -1 divided by the density ρ in g/cm 3 ) is more useful, and the attenuation law is written as
I = I o e -(μ/ρ)ρt Equation (1)
where I is the intensity of the radiation and t is the thickness. The product ρt is the significant parameter and the units (as with β and α particles) are mg/cm 2 , making the exponent in Equation (1) dimensionless.
Unlike charged particles, a certain percentage of gammas will always make it through the absorber, and it is useful to consider the half-value thickness of a given absorbing material for the gamma ray energies of interest. The half-value thicknesses are determined from Equation (1) using the linear attenuation or mass attenuation coefficients found in the references below. [6] Absorbers of these thicknesses attenuate the radiation reaching the detector by a factor of two and some of the common ones are tabulated below for Co-60 (1.33 and 1.17 MeV) and Cs-137 (662 keV).
Setting it Up
A Co-60 source (labeled #9) from the Phys 191 lab is the most convenient source for this demonstration in terms of strength (4 micro Ci as of 2016 ... half-life is 5.27 yrs). Position it approximately 2.5 inches in front of the Geiger-Muller tube. A 1/2" thick piece of lead cuts the count rate down by a factor of two and a 1.5" thick piece reduces the rate by a factor of ten.
Plastic, aluminum, steel, lead, and many other absorbers are available as needed. They range in thicknesses from hundreds of microns (foils) to several centimeters. The absorber is simply placed over the thin end-window of the G-M tube.
One doesn't really want to turn a simple demonstration into a lengthy laboratory exercise, so it's best to decide beforehand the salient features one wants to impress on the audience and use the appropriate absorbers and thicknesses to make the point.
AIP Physics Desk Reference , edited by E. Richard Cohen, David R. Lide, George L.. Trigg, (Springer, New York, 2003) G.F. Knoll, Radiation Detection and Measurement , 2nd ed, (Wiley, NY, 1989) G.W. Morgan, Some Practical Considerations in Radiation Shielding , Isotopes Division Circular B-4, (U.S. Atomic Energy Commission, Oak Ridge) CRC Handbook of Radioactive Nuclides , edited by Y. Wang, (Chemical Rubber Company, Ohio, 1969) A.H. Wapstra, G.J. Nijgh, and R. Van Lieshout, Nuclear Spectroscopy Tables , (North Holland, Amsterdam, 1959) X-ray Attenuation Coefficients from 10 keV to 100 MeV , National Bureau of Standards Circular No. 583 https://physics.nist.gov/PhysRefData/XrayMassCoef/tab3.html https://physics.nist.gov/PhysRefData/XrayMassCoef/tab2.html
[1] Indeed, in the early days of radiation measurement, alpha particle energies were measured indirectly by determining the absorber thickness equivalent to their mean range.
[2] The range is expressed in terms of (density)×(thickness), which is written as the mass/unit area of the absorber of a given thickness. Historically the units have been mg/cm 2 . Density·thickness (also sometimes referred to as mass·thickness) is a useful concept when discussing the energy loss of alphas and betas because, for absorber materials with similar neutron/proton ratios, a particle will encounter about the same number of electrons passing through absorbers of equal density·thickness. Therefore the stopping power and range, when expressed in these units, are roughly the same for materials that do not differ greatly in Z.
[3] The paper weighs 4.77 gm/sheet which gives it a density of 0.89 gm/cm 3 and a density·thickness of 7.9 mg/cm 2
[4] This rule of thumb is applicable only when E > 0.8 MeV. For other energy ranges, see Wang, p 912.
[5] Values are from Y. Wang ( reference ). Another rule-of-thumb is that the half-value range is approximately 1/7 of the maximum range but may vary between 1/5 and 1/10 (depending on beta energy and absorber density).
[6] The mass absorption coefficient also depends on the energy of the radiation. To calculate the half-value layer for a particular material and specific radiation energy, then one has to look up the "mass energy absorption coefficient" in the CRC Handbook or AIP Physics Desk Reference , or whichever reference is handy.
Demo Subjects
Newtonian Mechanics Fluid Mechanics Oscillations and Waves Electricity and Magnetism Light and Optics Quantum Physics and Relativity Thermal Physics Condensed Matter Astronomy and Astrophysics Geophysics Chemical Behavior of Matter Mathematical Topics
Key to Catalog Listings
Size : from small [S] (benchtop) to extra large [XL] (most of the hall) Setup Time : <10 min [t], 10-15 min [t+], >15 min [t++] /span> Rating : from good [★] to wow! [★★★★] or not rated [—]
Complete key to listings

- Science Notes Posts
- Contact Science Notes
- Todd Helmenstine Biography
- Anne Helmenstine Biography
- Free Printable Periodic Tables (PDF and PNG)
- Periodic Table Wallpapers
- Interactive Periodic Table
- Periodic Table Posters
- How to Grow Crystals
- Chemistry Projects
- Fire and Flames Projects
- Holiday Science
- Chemistry Problems With Answers
- Physics Problems
- Unit Conversion Example Problems
- Chemistry Worksheets
- Biology Worksheets
- Periodic Table Worksheets
- Physical Science Worksheets
- Science Lab Worksheets
- My Amazon Books
Alpha Particle – Definition, Symbol and Charge

An alpha particle is a particle consisting of two protons, two neutrons, and no electrons. Essentially, it is a helium-4 nucleus. Other names for alpha particles are alpha rays or alpha radiation. The symbol for an alpha particle is α, α 2+ , He 2+ , or 4 2 He 2+ . Usually, alpha particles result from alpha radioactive decay , but they form via other processes, too.
Alpha Particle Properties
Alpha particles have a net spin of zero. Their kinetic energy varies, with higher energy from larger nuclei (~7 MeV) and lower energy from smaller nuclei (~3 MeV). The average kinetic energy is around 5 MeV, with a velocity approximately 4% the speed of light . Because it lacks electrons, an alpha particle has a net electrical charge of 2+. However, the particles readily accept electrons from other matter and quickly become regular electrically neutral helium atoms ( 4 2 He).
Alpha particles are a form of ionizing radiation and can cause considerable damage to living tissue. However, alpha particles produced by radioactive decay have a lower penetration depth than, for example, beta or gamma radiation. Usually, all it takes is a few centimeters of air, a sheet of paper, or a few layers of skin to stop an alpha particle.
However, the alpha particles that form from ternary fission (as opposed to radioactive decay) are much more energetic and penetrate about three times further. Alpha particles that are cosmic rays are so energetic that they can pass through the human body or several meters of radiation shielding. Similarly, alpha particles produced in particles accelerators are highly energetic and display high penetration.
Sources of Alpha Particles
Most of the time, alpha particles come from radioactive decay of heavy atoms, such as uranium, thorium, actinium, radium, and transuranic elements. The smallest nucleus capable of alpha decay is beryllium-8. Alpha decay decreases the mass number of the parent nucleus by four nucleons (two protons and two neutrons ). Since the atomic number decreases by two, alpha decay is a form of transmutation that results in the formation of a new element.
A Z X → A-4 Z-2 Y + 4 2 He
Note that even though an ionizing helium nucleus results ( 4 2 He 2+ ), the “2+” usually is omitted in the written reaction. This is because nuclear reactions only show what’s going on with the atomic nucleus.
Other sources of alpha particles are ternary fission (when three charged particle results from fission instead of two) particle accelerators, cosmic rays, and nuclear reactions within the cores of stars. In everyday life, products that contain alpha emitters include tobacco smoke ( polonium ), some smoke detectors (americium), heart pacemakers (plutonium), and static eliminators (polonium). Rocks, soils, and water contain varying amounts of uranium, thorium, and actinium and their decay products.
Biological Effects of Alpha Particles
Alpha particles resulting from alpha decay mainly pose a risk when inhaled, ingested, or injected. This is because they only penetrate the outer layers of skin or the cornea of the eye. They can also enter through an open wound.
If alpha particles enter the body, they are so massive and reactive that they are the most destructive form of radiation. Significant doses of alpha radiation cause chromosome damage and radiation poisoning that can cause cancer or death. On average, alpha particles are between 20 times (ingested) and 1000 times (inhaled) more dangerous than beta particles or gamma radiation.
Alpha emitters also find use in the treatment of tumors. For example, radium -223 finds use in treating bone cancer and actinium-225 is used in treating prostate cancer. Alpha emitters used in cancer treatment tend to be radioisotopes with short half-lives. They either rely on their short effective range or else they migrate to specific tissues in the body.
Anti-Alpha Particle
As with other particles, there is an antimatter version of an alpha particle, which is the anti-alpha. An international team of researchers detected the anti-alpha using the STAR detector at Brookhaven National Laboratory in 2011. The experiment involved colliding gold ions moving nearly at the speed of light. At the time, the anti-alpha or antimatter helium-4 nucleus was the heaviest antimatter particle.
- Agakishiev, H.; et al. (STAR collaboration) (2011). “Observation of the antimatter helium-4 nucleus”. Nature . 473 (7347): 353–6. doi: 10.1038/nature10079
- Christensen, D. M.; Iddins, C. J.; Sugarman, S. L. (2014). “Ionizing radiation injuries and illnesses”. Emergency Medicine Clinics of North America . 32 (1): 245–65. doi: 10.1016/j.emc.2013.10.002
- Firestone, Richard B. (1999). Table of Isotopes . Coral M. Baglin (8th ed.). New York: Wiley. ISBN 0-471-35633-6.
- Krane, Kenneth S. (1988). Introductory Nuclear Physics. John Wiley & Sons. ISBN 978-0-471-80553-3.
- Little, John B.; Kennedy, Ann R.; McGandy, Robert B. (1985). “Effect of Dose Rate on the Induction of Experimental Lung Cancer in Hamsters by α Radiation”. Radiation Research. 103 (2): 293–9. doi: 10.2307/ 3576584
Related Posts
Alpha particles and alpha radiation: Explained
Alpha particles are also known as alpha radiation.
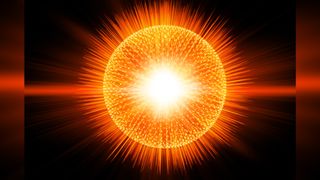
What are alpha particles?
Rutherford's gold foil experiment explained, what is alpha decay and how does it happen, alpha radiation charge and penetrating power, uses of alpha radiation, dangers of alpha radiation, additional resources.
Alongside beta particles, gamma rays , and neutrons, alpha particles are a type of radiation, also called alpha radiation. As with most forms of radiation, alpha particles are emitted from radioactive elements.
Alpha particles are relatively heavy and slow compared to their companions, so they pose little danger to humans unless ingested.
They are, however, frequently used in research — alpha particles were crucial in Ernest Rutherford's discovery of the atomic nucleus, which was the foundation of his atomic model.
Alpha particles are positively charged particles that comprise two protons, two neutrons, and zero electrons . A single particle's mass is 4 amu (6.642×10−4 g), according to Britannica Alpha particles are emitted from heavy radioactive elements (both naturally occurring and man-made), including uranium, radium, and plutonium. Because of this, these elements are also called alpha emitters.
An alpha particle is commonly represented by the symbol α, the Greek letter alpha for which the particle is named. It was the first type of nuclear radiation to be discovered, before beta particles and gamma rays. But because an alpha particle is identical to the nucleus of a helium-4 atom, it is sometimes represented as He2+, that is, a doubly ionized helium-4 atom.
Between 1898 and 1899, physicist Ernest Rutherford, who was studying radioactivity at Cambridge University in England, determined that there were at least two types of radiation , which he named alpha and beta. The alpha particle would lead to his discovery of the atomic nucleus — and help him develop the Rutherford atomic model, a radical shift in humanity's understanding of atoms .
In 1911, Rutherford officially published a paper declaring the existence of a positively charged nucleus at the center of an atom (though he didn't formally call it a nucleus at this point). Since 1907, Rutherford, Hans Geiger, and Ernest Marsden had been performing a series of Coulomb scattering experiments at the University of Manchester in England. Those experiments involved shooting alpha particles at thin gold foil, then observing where those particles went after colliding with the foil.
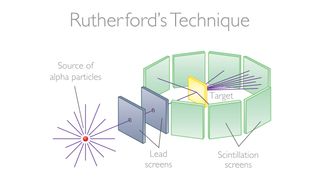
At the time, J. J. Thompson's "plum pudding" atomic model was the dominant theory of atomic structure — it suggested atoms were perfect spheres of positively charged material in which negative electrons floated about with relatively even distribution.
If that model were true, alpha particles would have passed through the foil in Rutherford's Coulomb experiments. But Rutherford and his colleagues observed that a few of the alpha particles bounced off the foil in different directions. Rutherford then theorized that atoms had a dense nucleus surrounded by orbiting electrons — the alpha particles went through the space between the electrons and bounced off the nucleus.
Alpha decay is the process by which alpha particles are formed , according to Britannica. Unstable radioactive elements called radionuclides emit particles from their nuclei to become more stable, transforming from the original element into a new one. Those emissions are radiation — in the case of alpha decay, alpha particles are emitted from the nuclei of heavy radioactive elements.
Alpha radiation has a positive charge of two. Of the main types of radiation, alpha particles are the heaviest and slowest, with a mass of 4 amu and ejection speeds of approximately 12,400 miles per second (20,000,000 km per second) according to the Australian Radiation Protection and Nuclear Safety Agency .
— The double-slit experiment: Is light a wave or a particle?
— Tachyons: Facts about these faster-than-light particles
— What is dark matter?
Despite being highly energetic, alpha particles expend most of that energy immediately after emission, so they do not travel farther than a few inches at most. They also have extremely low penetrating power — they cannot penetrate a human's epidermis, or outer layer of skin. Even a piece of paper is enough to block an alpha particle per the United States Nuclear Regulatory Committee .
Commercially, alpha radiation is primarily used in smoke detectors (smoke reduces the alpha particles in the detector, triggering the alarm) and static eliminators (alpha ionizers).
There is also ongoing research into developing alpha-particle therapy to treat cancer — clinical trials have found some success in treating metastatic, castration-resistant prostate cancer. For research purposes, alpha particles are used as projectiles, as in the case of Rutherford's gold foil experiment.
Alpha radiation is not dangerous to humans externally due to its low penetrating power; alpha particles cannot penetrate your skin. They can, however, cause damage to your cornea .
The real danger occurs inside the body. If an alpha emitter (that is, a radioactive element) enters your body via ingestion, inhalation, a wound, or any other means, great damage could be done internally to living tissue.
Read more about Rutherford's work with alpha particles in this online exhibition by the American Institute of Physics' Center for History. You can also learn more on his biography page from the Nobel Foundation — Rutherford was awarded the Nobel Prize in Chemistry in 1908 "for his investigations into the disintegration of the elements, and the chemistry of radioactive substances."
Bibliography
Australian Radiation Protection and Nuclear Safety Agency ( ARPANSA ), "Alpha particles."
Britannica, "Alpha decay."
Britannica , "Alpha particle."
United States Environmental Protection Agency (EPA), "Radiation Basics."
United States Nuclear Regulatory Commission (USNRC), " Radiation Basics. "
Center for History, American Institute of Physics, " Rutherford's Nuclear World ."
Join our Space Forums to keep talking space on the latest missions, night sky and more! And if you have a news tip, correction or comment, let us know at: [email protected].
Get the Space.com Newsletter
Breaking space news, the latest updates on rocket launches, skywatching events and more!
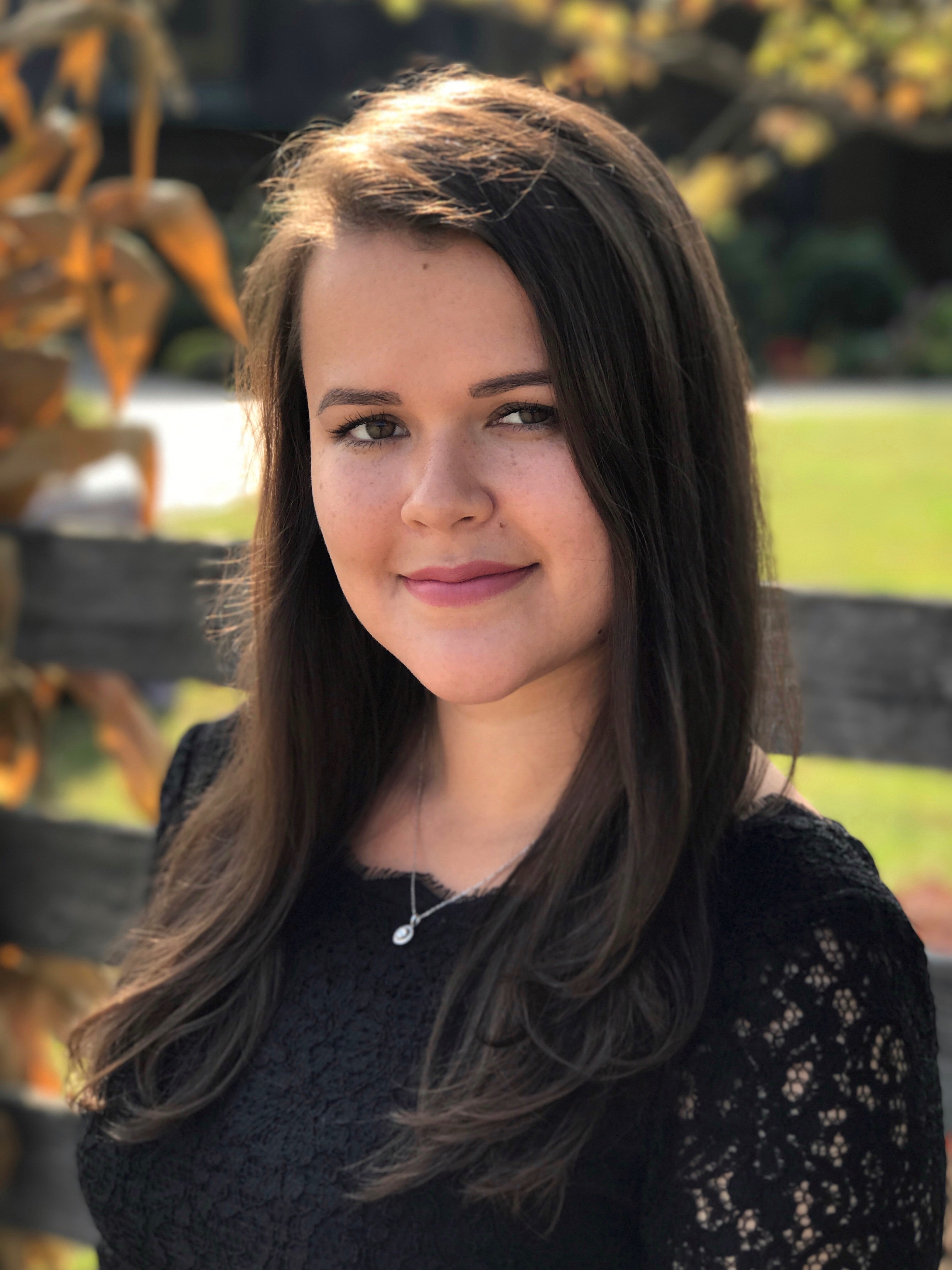
Space.com contributing writer Stefanie Waldek is a self-taught space nerd and aviation geek who is passionate about all things spaceflight and astronomy. With a background in travel and design journalism, as well as a Bachelor of Arts degree from New York University, she specializes in the budding space tourism industry and Earth-based astrotourism. In her free time, you can find her watching rocket launches or looking up at the stars, wondering what is out there. Learn more about her work at www.stefaniewaldek.com .
Car-size asteroid gives Earth a super-close shave with flyby closer than some satellites
SpaceX launches advanced weather satellite for US Space Force (video)
Saturn's ocean moon Enceladus is able to support life − my research team is working out how to detect extraterrestrial cells there
Most Popular
- 2 Why is it so hard to send humans back to the moon?
- 3 Eclipse expert Jamie Carter wins media award for extensive solar eclipse coverage
- 4 'Devil Comet' 12P/Pons-Brooks reaches peak brightness tonight. Here's how to see it
- 5 Cosmonaut Muhammed Faris, first Syrian in space, dies at 72
Alpha Particle
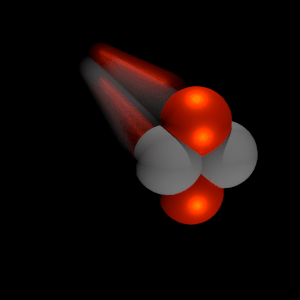
Interaction of Alpha Particles with Matter
Since the electromagnetic interaction extends over some distance, it is unnecessary for alpha particles to collide with an atom directly. They can transfer energy simply by passing close by . Alpha particles interact with matter primarily through coulomb forces between their positive charge and the negative charge of the electrons from atomic orbitals. In general, the alpha particles (like other charged particles) transfer energy mostly by:
- Excitation. The charged particle can transfer energy to the atom, raising electrons to higher energy levels.
- Ionization. Ionization can occur when the charged particle has enough energy to remove an electron. This results in the creation of ion pairs in surrounding matter.
The creation of pairs requires energy, which is lost from the kinetic energy of the alpha particle, causing it to decelerate . The positive ions and free electrons created by the passage of the alpha particle will then reunite, releasing energy in the form of heat (e.g.,, vibrational energy or rotational energy of atoms). There are considerable differences in the ways of energy loss and scattering between the passage of light-charged particles such as positrons and electrons and heavy charged particles such as fission fragments, alpha particles, muons. Most of these differences are based on the different dynamics of the collision process. In general, when a heavy particle collides with a much lighter particle (electrons in the atomic orbitals), the laws of energy and momentum conservation predict that only a small fraction of the massive particle’s energy can be transferred to the less massive particle. The actual amount of transferred energy depends on how closely the charged particles pass through the atom, and it also depends on restrictions from the quantization of energy levels.
See also: Interaction of Heavy Charged Particles with Matter
Stopping Power – Bethe Formula
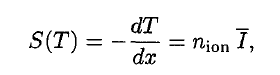
Where T is the kinetic energy of the charged particle, n ion is the number of electron-ion pairs formed per unit path length, and I denotes the average energy needed to ionize an atom in the medium. For charged particles, S increases as the particle velocity decreases . The classical expression that describes the specific energy loss is known as the Bethe formula . Hans Bethe found the non-relativistic formula in 1930. Hans Bethe also found the relativistic version (see below) in 1932.

In this expression, m is the rest mass of the electron, β equals v/c, which expresses the particle’s velocity relative to the speed of light, γ is the Lorentz factor of the particle, Q equals to its charge, Z is the atomic number of the medium and n is the density of the atoms in the volume. For non-relativistic particles (heavy charged particles are mostly non-relativistic), dT/dx is dependent on 1/v 2 . This can be explained by the greater time the charged particle spends in the negative field of the electron when the velocity is low.
The stopping power of most materials is very high for heavy-charged particles, and these particles have very short ranges. For example, a 5 MeV alpha particle’s range is approximately 0,002 cm in aluminium alloy. Most alpha particles can be stopped by an ordinary sheet of paper or living tissue. Therefore the shielding of alpha particles does not pose a difficult problem. On the other hand, alpha radioactive nuclides can lead to serious health hazards when ingested or inhaled (internal contamination).
Bragg Curve
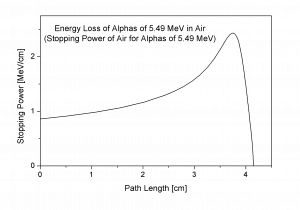
The Bragg curve is typical for alpha particles and other heavy-charged particles and describes the energy loss of ionizing radiation during travel through matter. This curve is typical of the Bragg peak , which results from 1/v 2 dependency of the stopping power. This peak occurs because the cross-section of interaction increases immediately before the particle comes to rest. The charge remains unchanged for most of the track, and the specific energy loss increases according to the 1/v 2 . Near the end of the track, the charge can be reduced through electron pickup, and the curve can fall off.
The Bragg curve also differs somewhat due to the effect of straggling . For a given material, the range will be nearly the same for all particles of the same kind with the same initial energy. Because the details of the microscopic interactions undergone by any specific particle vary randomly, a small variation in the range can be observed. This variation is called straggling, and it is caused by the statistical nature of the energy loss process, which consists of many individual collisions.
This phenomenon, described by the Bragg curve, is exploited in particle therapy of cancer because this allows concentrating the stopping energy on the tumor while minimizing the effect on the surrounding healthy tissue.
See previous:
Fundamental Particles
Beta Particle
Applications
- MiniPIX EDU
- Education Kit
- Experiments
How far can Alpha Particles Travel?
Stage, camera holder, source holder, polonium-210, Minipix-EDU
- Min Level: 0
- Max Level: 100
- Measurement Mode: Tracking
- Frames: 100
- Exposure: 1 s
- Sum: uncheck
- Color Map: Hot
- Mount the MiniPix EDU camera and the holder with polonium on the stage.
- Keep the camera and the source as close as possible and click on the play button.
- Next, shift the source to 2 cm and collect the data. Similarly, collect the data for 3 cm and 4 cm.
- As explained earlier, alpha particles are the heaviest among the three and are more ionizing. They quickly lose their energy in the air and thus have a very short mean linear range.
- The alpha particles from polonium-210 have only ~4.5 cm of range.

Robotic Imaging

- Terms of Use
- Have your say (Opens in a new tab/window)
- What is ionising radiation?
- Achived - What is ionising radiation?
- What is background radiation?
- What is non-ionising radiation?
- Units for measuring radiation
- Glossary of terms
- Sun protection
- Smart meters
- Mobile phone base stations
- Radioactive waste
- More radiation sources
- Occupational exposure
- Ultraviolet radiation monitoring
- Personal Radiation Monitoring Service
- Australian National Radiation Dose Register (ANRDR)
- The Australian National Radiation Dose Register
- Buyer's guide for sun protection
- Ultraviolet services
- Australian Clinical Dosimetry Service
- Radioanalytical services
- Calibration
- Occupational Radiation Exposure (ORE) for medical facilities
- Practical reference dosimetry course
- Radiation Protection of the Patient
- Radiation safety training courses
- Radiation meter hire - order form
- Non-medical import permit details
- Medical import permit details
- Export permit details
- Our regulatory services
- State & territory regulators
- Regulatory integrity
- International best practice
- Information for licence applicants
- Information for licence holders
- Australian Radiation Incident Register
- Holistic safety
- Radioactive waste disposal and storage
- Security of radioactive material
- Transport of radioactive material
- Publications program
- Radiation Protection Series
- Radiation Health Series
- National Directory for Radiation Protection
- Regulatory Guides
- Regulatory forms
- New radiation equipment
- Radiation literature survey
- Survey of residential radio wave exposure 2022
- Brain tumour study
- National Diagnostic Reference Level Service (NDRLS)
- Wi-fi in schools measurement study
- ARPANSA environmental EME reports
- Base station survey
- Survey of residential power frequency magnetic fields
- Australian national diagnostic reference levels for MDCT - ADULT
- Radiofrequency Electromagnetic Energy and Health: Research Needs (TR 178)
- Australasian Radioanalytical Laboratory Network
- Early notification and assistance conventions
- Nuclear-powered vessel visit planning
- Radiation Emergency Medical Preparedness and Assistance Network
- Australian Radon Action Plan
- ARPANSA EME Program Action Plan 2020-2024
- Electromagnetic Energy Research
- Research Framework
- National collaboration
- National uniformity
- International collaboration
- Organisational structure
- Radiation Health and Safety Advisory Council
- Radiation Health Committee
- Nuclear Safety Committee
- Roles and expectations for advisory bodies
- Audit and Risk Committee
- Our service charter
- Corporate plan
- Planning and accountability
- Regulator Performance Framework
- Work health and safety policy statement
- Annual Report series
- Quarterly Report series
- ARPANSA Research Strategy
- Significant regulatory activities
- Current vacancies
- Applying for a job
- Working with ARPANSA
- ARPANSA Graduate Program
- Access to information
- Child Safe Framework compliance statement
- Executive remuneration reporting 2017-18
- Freedom of information requests
- Gifts and benefit register
- Information Publication Scheme
- Legal services expenditure reporting
- Senate Procedural Order of continuing effect No. 12
- Senate Order on Entity Contracts
- Public Interest Disclosure Scheme
- Reserve Bank of Australia's terms and conditions for ARPANSA
- Privacy Policy
- Quality Policy
- Regulatory Activities Policy
- Work Health and Safety Policy
- Determining breaches of the APS Code of Conduct and deciding sanctions
Alpha particles
Alpha particles are relatively slow and heavy compared with other forms of nuclear radiation., what are alpha particles.
Alpha particles ( a ) are composite particles consisting of two protons and two neutrons tightly bound together (Figure 1). They are emitted from the nucleus of some radionuclides during a form of radioactive decay , called alpha-decay. An alpha-particle is identical to the nucleus of a normal (atomic mass four) helium atom i.e. a doubly ionised helium atom.
Alpha particles (also termed alpha radiation or alpha rays) was the first nuclear radiation to be discovered, beta particles and gamma rays were identified soon after.

What are the properties of alpha particles?
Alpha particles are relatively slow and heavy compared with other forms of nuclear radiation. The particles travel at 5 to 7 % of the speed of light or 20,000,000 metres per second and has a mass approximately equivalent to 4 protons.
Alpha particles, because they are highly ionising, are unable to penetrate very far through matter and are brought to rest by a few centimetres of air or less than a tenth of a millimetre of biological tissue (Figure 2).
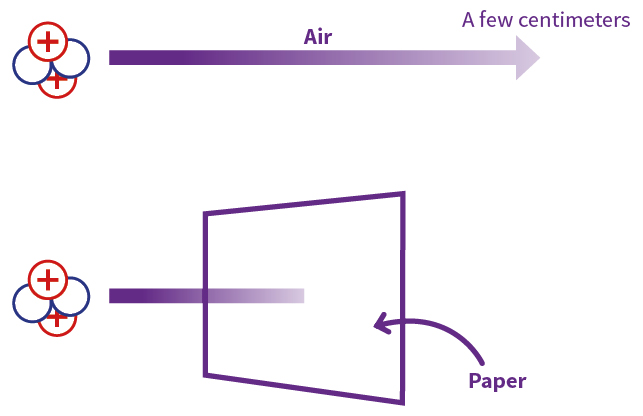
What are the health effects of exposure to alpha particles?
Alpha particles are highly ionising because of their double positive charge, large mass (compared to a beta particle) and because they are relatively slow. They can cause multiple ionisations within a very small distance. This gives them the potential to do much more biological damage for the same amount of deposited energy.
Alpha particles can't penetrate the normal layer of dead cells on the outside of our skin but can damage the cornea of the eye. Alpha-particle radiation is normally only a safety concern if the radioactive decay occurs from an atom that is already inside the body or a cell. Alpha-particle emitters are particularly dangerous if inhaled, ingested, or if they enter a wound.
What are some common sources of alpha particles?
Many alpha emitters occur naturally in the environment. For example, alpha particles are given off by radionuclides such as uranium-238, radium-226, and other members of the naturally occurring uranium, thorium and actinium decay series which are present in varying amounts in nearly all rocks, soils, and water.
Artificially produced sources of alpha particles include the radioisotopes of elements such as plutonium, americium, curium and californium. These are generally produced in a nuclear reactor through the absorption of neutrons by various uranium radioisotopes.
What are some uses of alpha particles?
Alpha particles have low penetrating power but this still provides a range of useful applications:
- smoke detectors – americium-241 is commonly used in ionising smoke detectors. Smoke that enters the detector reduces the amount of alpha particles that are detected and triggers the alarm
- static eliminators typically use alpha particles from polonium-210 to remove static charges from equipment
- radioisotope thermoelectric generators use alpha particle decay from plutonium-238 to generate heat which is converted to electricity, commonly used in space probes
- some alpha emitters are being investigated for their potential use in unsealed source radiotherapy to treat cancer.
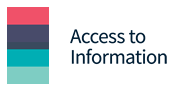
PhysicsOpenLab Modern DIY Physics Laboratory for Science Enthusiasts
Alpha particles range & bragg curve.
September 10, 2017 Alpha Spectroscopy , English Posts 21,081 Views

The energy of alpha particles varies, with higher energy alpha particles being emitted from larger nuclei, but most alpha particles have energies of between 3 and 7 MeV , corresponding to extremely long and extremely short half-lives of alpha-emitting nuclides, respectively.
This energy is a substantial amount of energy for a single particle, but their high mass means alpha particles have a lower speed (with a typical kinetic energy of 5 MeV; the speed is 15,000 km/s, which is 5% of the speed of light) than any other common type of radiation (β particles, neutrons, etc.). Because of their charge and large mass , alpha particles are easily absorbed by materials, and they can travel only a few centimeters in air . They can be absorbed by tissue paper or the outer layers of human skin (about 40 μm, equivalent to a few cells deep).

Some Theory
The interaction of charged particles or photons with matter is electromagnetic and results either in a gradual reduction of energy of the incoming particle (with a change in its direction) or in the absorption of the photon. Particles such as nuclei, protons, neutrons and mesons are subject to a nuclear interaction as well, which is, however, of much shorter range than the electromagnetic one. The nuclear interaction may become predominant only when the particles have enough energy to overcome Coulomb-barrier effects.
Heavy charged particles lose energy through collisions with the atomic electrons of the material, while electrons lose energy both through collisions and through radiation when their trajectory is altered by the field of a nucleus ( bremsstrahlung radiation). Therefore fast charged particles moving through matter interact with the electrons of atoms in the material. The interaction excites or ionizes the atoms, leading to an energy loss of the traveling particle.
The mean energy loss per distance traveled of swift charged particles (protons, alpha particles, atomic ions) traversing matter (or alternatively the stopping power of the material) is described by the Bethe formula . For electrons the energy loss is slightly different due to their small mass (requiring relativistic corrections) and their indistinguishability, and since they suffer much larger losses by Bremsstrahlung, terms must be added to account for this. For low energies, i.e., for small velocities of the particle, the Bethe formula reduces to :

Range of a Charged Particle
Both charged and uncharged particles lose energy while passing through matter, but stopping power describes only the energy loss of charged particles. The stopping power depends on the type and energy of the radiation and on the properties of the material it passes. Since the production of an ion pair (usually a positive ion and a (negative) electron) requires a fixed amount of energy (for example, 33.97 eV in dry air ), the density of ionization is proportional to the stopping power. The stopping power of the material is numerically equal to the loss of energy E per unit path length, x :
The force usually increases toward the end of range and reaches a maximum, the Bragg peak , shortly before the energy drops to zero. The curve that describes the force as function of the material depth is called the Bragg curve . This is of great practical importance for radiation therapy. The mean range of the particles can be calculated by integrating the reciprocal stopping power over energy:
From the above equation the mean range can be calculated and it results that it is proportional to the mass of the incoming particle, inversely proportional to the square of its charge, and inversely proportional to the electron density of the stopping material.
The Experiment
The experiment aims to measure the range of alpha particles in a dense material such as silver, evaluate the energy drop and plot the Bragg curve.
The experiment on measuring absorbance of alpha particles in matter is based on the alpha spectrometer described in the post DIY Alpha Spectrometer . The instrument is based on a PIPS solid state sensor with active area of 100 mm 2 , the sensor is placed inside a vacuum chamber. The signal processing chain includes a charge sensitive preamplifier followed by a shaper amplifier. The signal, properly amplified and shaped, is acquired by the audio ADC of the PC and processed by the multichannel Theremino MCA analyzer.
A Po210 source and the detector are both housed inside a vacuum chamber at fixed-distance. The alpha particle source is screened with a growing number of extremely thin silver sheets (the thickness of the silver sheet is estimated at 0.5 μm ). Depending on the thickness of total silver layer we measure:
- number of particles counted by the detector
- energy spectrum of particles, with a measurement of the particles mean energy
The image below shows the source and the silver sheets used as a screen.

The image below shows the detector and shielded source for a measurement.

Range Measures
In the range measure, the same number of alpha particles should be reaching the detector until the number of silver foil placed between the source and the detector reaches the range of alpha particles. Further increasing of the number of silver layers causes the counting rate of the detector should abruptly fall to 0. We point out that the relative position of source and detector is not changed and therefore the solid angle “seen” by the detector remains constant. The only variation is the increase in the scattering by the silver sheets that may result in a small particle loss from the beam. The results of this measure are shown in the graph below. 27 sheets of silver leaf were used before counting zero. From the graph below you get a value of 12 μm for the range.

Energy Measures
As far the energy measurements are concerned, the image below shows the distribution of the detector pulse heights as obtained from our alpha spectrometer. Each peak corresponds to a different number of silver leafs. The first is obtained without silver sheets and corresponds to the Po210 alpha particle energy, the second corresponds to 5 layers, the third 10 layers and the fourth corresponds to 15 layers. The more are the silver layers the lower is the energy but the total particle count (area under the peak) remains constant. The thicker is the stopping material the wider is the energy peak : this is the energy straggling.

Taking energy data for each number of layers, from 0 to 27 we obtain the plot below which shows the residual energy of a alpha particle when it reaches the detector as a function of silver thickness. The points are fitted with a polynomial curve.
The second plot shows the curve obtained from the derivative of the energy curve, thus it gives the energy loss per unit length. It is called the “Bragg curve” .

The experimental results for the Bragg curve are in good agreement with the theory : the obtained curve shows a 1/E dependence as predicted by the equations and the peak of the energy loss, just before it drops to 0, is evident : as the particle reaches the end of its range the energy loss dE/dx reaches a maximum and then it drops rapidly to 0.
If you liked this post you can share it on the “social” Facebook , Twitter or LinkedIn with the buttons below. This way you can help us! Thank you !
If you like this site and if you want to contribute to the development of the activities you can make a donation, thank you !
Tags Alpha Spectrometry

CALL for Donations
The PhysicsOpenLab adventure has lasted for several years now. They were beautiful years in which …
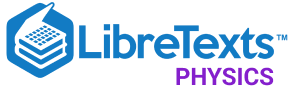
- school Campus Bookshelves
- menu_book Bookshelves
- perm_media Learning Objects
- login Login
- how_to_reg Request Instructor Account
- hub Instructor Commons
- Download Page (PDF)
- Download Full Book (PDF)
- Periodic Table
- Physics Constants
- Scientific Calculator
- Reference & Cite
- Tools expand_more
- Readability
selected template will load here
This action is not available.
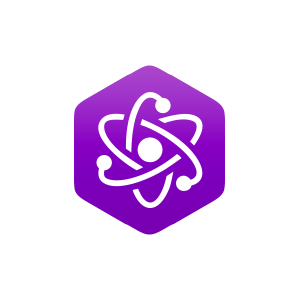
21.4: Motion of a Charged Particle in a Magnetic Field
- Last updated
- Save as PDF
- Page ID 15649
learning objectives
- Compare the effects of the electric and the magnetic fields on the charged particle
Electric vs. Magnetic Forces
Force due to both electric and magnetic forces will influence the motion of charged particles. However, the resulting change to the trajectory of the particles will differ qualitatively between the two forces. Below we will quickly review the two types of force and compare and contrast their effects on a charged particle.
Electrostatic Force and Magnetic Force on a Charged Particle
Recall that in a static, unchanging electric field E the force on a particle with charge q will be:
\[\mathrm { F } = \mathrm { qE }\]
Where F is the force vector, q is the charge, and E is the electric field vector. Note that the direction of F is identical to E in the case of a positivist charge q, and in the opposite direction in the case of a negatively charged particle. This electric field may be established by a larger charge, Q, acting on the smaller charge q over a distance r so that:
\[\mathrm { E } = \left| \frac { \mathrm { F } } { \mathrm { q } } \right| = \mathrm { k } \left| \frac { \mathrm { qQ } } { \mathrm { qr } ^ { 2 } } \right| = \mathrm { k } \frac { | \mathrm { Q } | } { \mathrm { r } ^ { 2 } }\]
It should be emphasized that the electric force F acts parallel to the electric field E . The curl of the electric force is zero, i.e.:
\[\nabla \times \mathrm { E } = 0\]
A consequence of this is that the electric field may do work and a charge in a pure electric field will follow the tangent of an electric field line .
In contrast, recall that the magnetic force on a charged particle is orthogonal to the magnetic field such that:
\[\mathrm { F } = \mathrm { qv } \times \mathrm { B } = \mathrm { q } \mathrm { vB } \sin \theta\]
where B is the magnetic field vector, v is the velocity of the particle and θ is the angle between the magnetic field and the particle velocity. The direction of F can be easily determined by the use of the right hand rule.
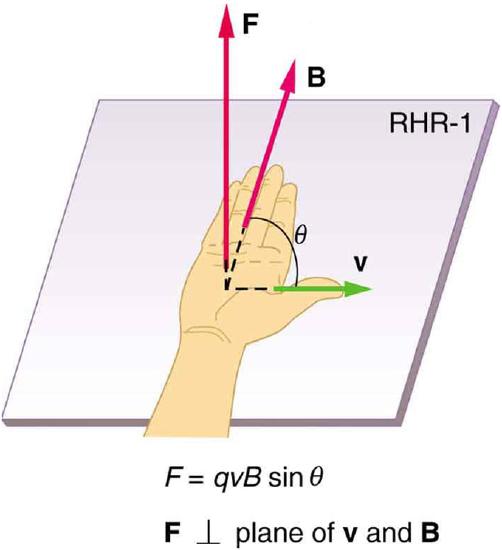
Right Hand Rule : Magnetic fields exert forces on moving charges. This force is one of the most basic known. The direction of the magnetic force on a moving charge is perpendicular to the plane formed by v and B and follows right hand rule–1 (RHR-1) as shown. The magnitude of the force is proportional to q, v, B, and the sine of the angle between v and B.
If the particle velocity happens to be aligned parallel to the magnetic field, or is zero, the magnetic force will be zero. This differs from the case of an electric field, where the particle velocity has no bearing, on any given instant, on the magnitude or direction of the electric force.
The angle dependence of the magnetic field also causes charged particles to move perpendicular to the magnetic field lines in a circular or helical fashion, while a particle in an electric field will move in a straight line along an electric field line.
A further difference between magnetic and electric forces is that magnetic fields do not net work, since the particle motion is circular and therefore ends up in the same place. We express this mathematically as:
\[\mathrm { W } = \oint \mathrm { B } \cdot \mathrm { dr } = 0\]
Lorentz Force
The Lorentz force is the combined force on a charged particle due both electric and magnetic fields, which are often considered together for practical applications. If a particle of charge q moves with velocity v in the presence of an electric field E and a magnetic field B , then it will experience a force:
\[\mathrm { F } = \mathrm { q } [ \mathrm { E } + \mathrm { vB } \sin \theta ]\]
Electric and Magnetic Field Lines
We mentioned briefly above that the motion of charged particles relative to the field lines differs depending on whether one is dealing with electric or magnetic fields. There are some notable differences between how electric and magnetic field lines are conceptualized. The electric field lines from a positive isolated charge are simply a sequence of evenly-spaced, radially directed lines pointed outwards from the charge. In the case of a negative charge, the direction of the field is reversed. The electric field is directed tangent to the field lines. Of course, we imagine the field lines are more densely packed the larger the charges are. One can see clearly that the curl of the electric force is zero.
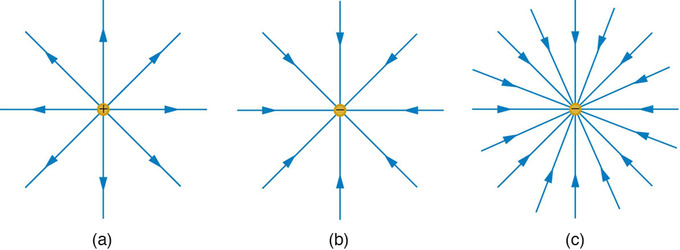
Electric Field Generated by Point Charges : The electric field surrounding three different point charges: (a) A positive charge; (b) a negative charge of equal magnitude; (c) a larger negative charge.
If multiple charges are involved, field lines are generated on positive charges, and terminate on negative ones.
In the case of magnets, field lines are generated on the north pole (+) and terminate on the south pole (-) – see the below figure. Magnetic ‘charges’, however, always come in pairs – there are no magnetic monopoles (isolated north or south poles). The curl of a magnetic field generated by a conventional magnet is therefore always non zero. Charged particles will spiral around these field lines, as long as the particles have some non-zero component of velocity directed perpendicular to the field lines.
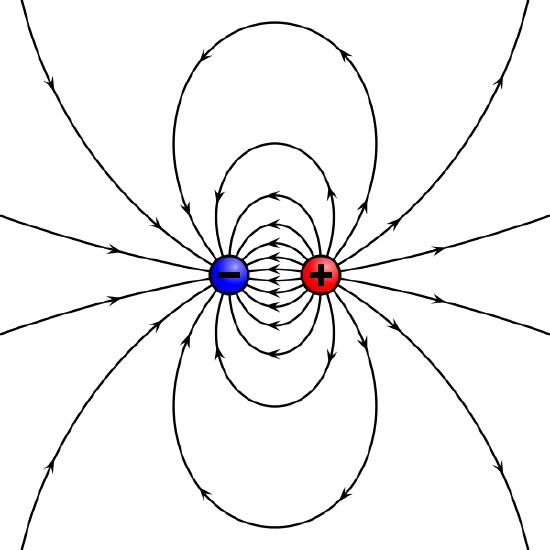
Magnetic Pole Model : The magnetic pole model: two opposing poles, North (+) and South (−), separated by a distance d produce an H-field (lines).
A magnetic field may also be generated by a current with the field lines envisioned as concentric circles around the current-carrying wire.The magnetic force at any point in this case can be determined with the right hand rule, and will be perpendicular to both the current and the magnetic field.
Constant Velocity Produces a Straight-Line
If a charged particle’s velocity is parallel to the magnetic field, there is no net force and the particle moves in a straight line.
- Identify conditions required for the particle to move in a straight line in the magnetic field
Constant Velocity Produces Straight-Line Motion
Recall Newton’s first law of motion. If an object experiences no net force, then its velocity is constant: the object is either at rest (if its velocity is zero), or it moves in a straight line with constant speed (if its velocity is nonzero).
There are many cases where a particle may experience no net force. The particle could exist in a vacuum far away from any massive bodies (that exert gravitational forces) and electromagnetic fields. Or there could be two or more forces on the particle that are balanced such that the net force is zero. This is the case for, say, a particle suspended in an electric field with the electric force exactly counterbalancing gravity.
If the net force on a particle is zero, then the acceleration is necessarily zero from Newton’s second law: F=ma. If the acceleration is zero, any velocity the particle has will be maintained indefinitely (or until such time as the net force is no longer zero). Because velocity is a vector, the direction remains unchanged along with the speed, so the particle continues in a single direction, such as with a straight line.
Charged Particles Moving Parallel to Magnetic Fields
The force a charged particle “feels” due to a magnetic field is dependent on the angle between the velocity vector and the magnetic field vector B . Recall that the magnetic force is:
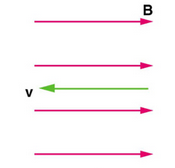
Zero Force When Velocity is Parallel to Magnetic Field : In the case above the magnetic force is zero because the velocity is parallel to the magnetic field lines.
\[\mathrm { F } = \mathrm { q } \mathrm { v } \mathrm { B } \sin \theta\]
If the magnetic field and the velocity are parallel (or antiparallel), then sinθ equals zero and there is no force. In this case a charged particle can continue with straight-line motion even in a strong magnetic field. If is between 0 and 90 degrees, then the component of v parallel to B remains unchanged.
Circular Motion
Since the magnetic force is always perpendicular to the velocity of a charged particle, the particle will undergo circular motion.
- Describe conditions that lead to the circular motion of a charged particle in the magnetic field
Circular Motion of a Charged particle in a Magnetic Field
Magnetic forces can cause charged particles to move in circular or spiral paths. Particle accelerators keep protons following circular paths with magnetic force. Cosmic rays will follow spiral paths when encountering the magnetic field of astrophysical objects or planets (one example being Earth’s magnetic field). The bubble chamber photograph in the figure below shows charged particles moving in such curved paths. The curved paths of charged particles in magnetic fields are the basis of a number of phenomena and can even be used analytically, such as in a mass spectrometer. shows the path traced by particles in a bubble chamber.
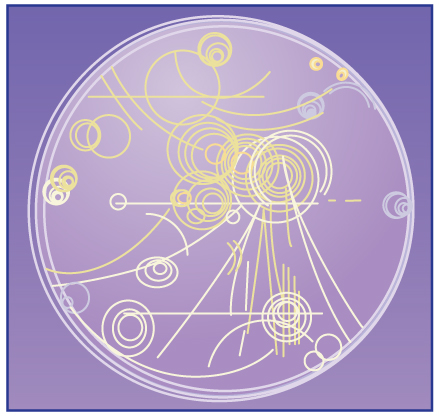
Bubble Chamber : Trails of bubbles are produced by high-energy charged particles moving through the superheated liquid hydrogen in this artist’s rendition of a bubble chamber. There is a strong magnetic field perpendicular to the page that causes the curved paths of the particles. The radius of the path can be used to find the mass, charge, and energy of the particle.
So, does the magnetic force cause circular motion? Magnetic force is always perpendicular to velocity, so that it does no work on the charged particle. The particle’s kinetic energy and speed thus remain constant. The direction of motion is affected, but not the speed. This is typical of uniform circular motion. The simplest case occurs when a charged particle moves perpendicular to a uniform B-field, such as shown in. (If this takes place in a vacuum, the magnetic field is the dominant factor determining the motion. ) Here, the magnetic force (Lorentz force) supplies the centripetal force
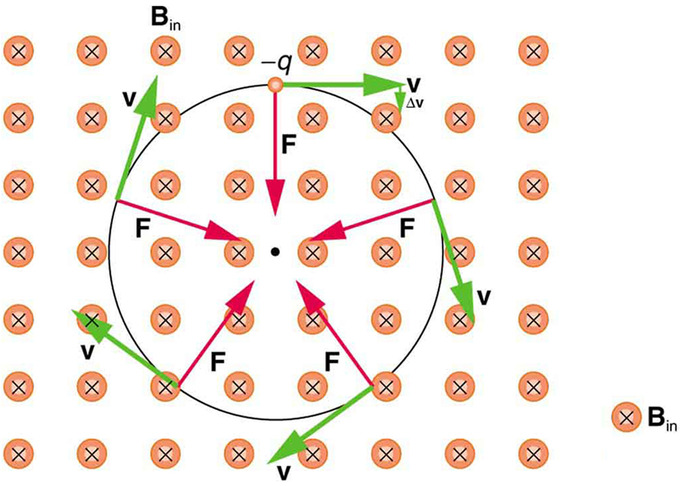
Circular Motion of Charged Particle in Magnetic Field : A negatively charged particle moves in the plane of the page in a region where the magnetic field is perpendicular into the page (represented by the small circles with x’s—like the tails of arrows). The magnetic force is perpendicular to the velocity, and so velocity changes in direction but not magnitude. Uniform circular motion results.
\[\mathrm { F } _ { \mathrm { c } } = \dfrac { \mathrm { mv } ^ { 2 } } { \mathrm { r } }\]
Noting that
\[\sin \theta = 1\]
we see that
\[\mathrm { F } = \mathrm { qvB }\]
The Lorentz magnetic force supplies the centripetal force, so these terms are equal:
\[\mathrm { qvB } = \dfrac { \mathrm { mv } ^ { 2 } } { \mathrm { r } }\]
solving for r yields
\[\mathrm { r } = \dfrac { \mathrm { m } \mathrm { v } } { \mathrm { qB } } \]
Here, r , called the gyroradius or cyclotron radius, is the radius of curvature of the path of a charged particle with mass m and charge q , moving at a speed v perpendicular to a magnetic field of strength B . In other words, it is the radius of the circular motion of a charged particle in the presence of a uniform magnetic field. If the velocity is not perpendicular to the magnetic field, then v is the component of the velocity perpendicular to the field. The component of the velocity parallel to the field is unaffected, since the magnetic force is zero for motion parallel to the field. We’ll explore the consequences of this case in a later section on spiral motion.
A particle experiencing circular motion due to a uniform magnetic field is termed to be in a cyclotron resonance . The term comes from the name of a cyclic particle accelerator called a cyclotron, showed in. The cyclotron frequency (or, equivalently, gyrofrequency) is the number of cycles a particle completes around its circular circuit every second and can be found by solving for v above and substituting in the circulation frequency so that
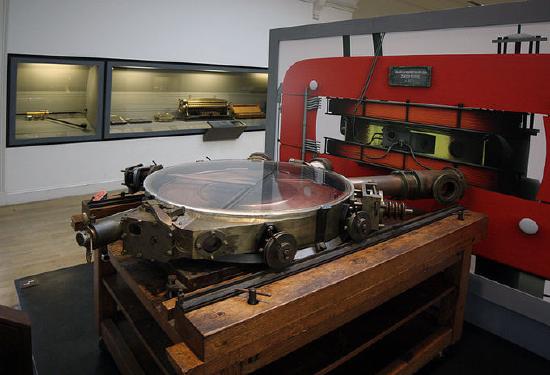
Cyclotron : A French cyclotron, produced in Zurich, Switzerland in 1937
\[\mathrm { f } = \dfrac { \mathrm { v } } { 2 \pi \mathrm { r } }\]
\[\mathrm{ f } = \dfrac { \mathrm { qB } } { 2 \pi \mathrm { m } } \]
The cyclotron frequency is trivially given in radians per second by
\[\omega = \dfrac { \mathrm{q} \mathrm { B } } { \mathrm { m } }\]
Helical Motion
Helical motion results when the velocity vector is not perpendicular to the magnetic field vector.
- Describe conditions that lead to the helical motion of a charged particle in the magnetic field
In the section on circular motion we described the motion of a charged particle with the magnetic field vector aligned perpendicular to the velocity of the particle. In this case, the magnetic force is also perpendicular to the velocity (and the magnetic field vector, of course) at any given moment resulting in circular motion. The speed and kinetic energy of the particle remain constant, but the direction is altered at each instant by the perpendicular magnetic force. quickly reviews this situation in the case of a negatively charged particle in a magnetic field directed into the page.
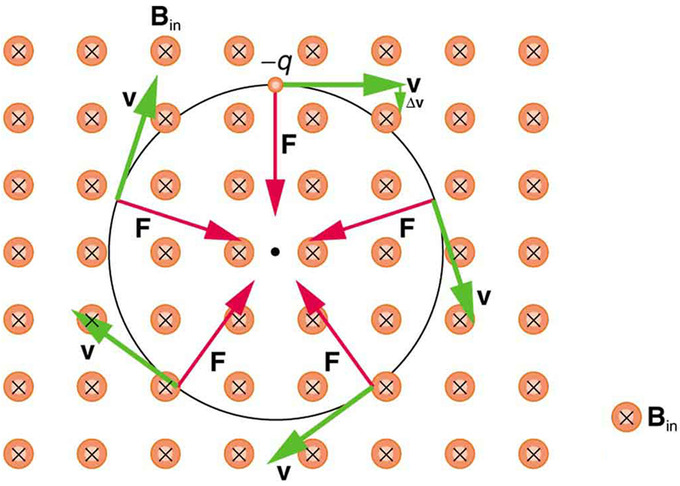
What if the velocity is not perpendicular to the magnetic field? Then we consider only the component of v that is perpendicular to the field when making our calculations, so that the equations of motion become:
\[\mathrm { F } _ { \mathrm { c } } = \dfrac { \mathrm { m } \mathrm { v } _ { \perp } ^ { 2 } } { \mathrm { r } }\]
\[\mathrm { F } = \mathrm { q } \mathrm { vB } \sin \theta = \mathrm { qv } _ { \perp } \mathrm { B }\]
The component of the velocity parallel to the field is unaffected, since the magnetic force is zero for motion parallel to the field. This produces helical motion (i.e., spiral motion) rather than a circular motion.
shows how electrons not moving perpendicular to magnetic field lines follow the field lines. The component of velocity parallel to the lines is unaffected, and so the charges spiral along the field lines. If field strength increases in the direction of motion, the field will exert a force to slow the charges (and even reverse their direction), forming a kind of magnetic mirror.
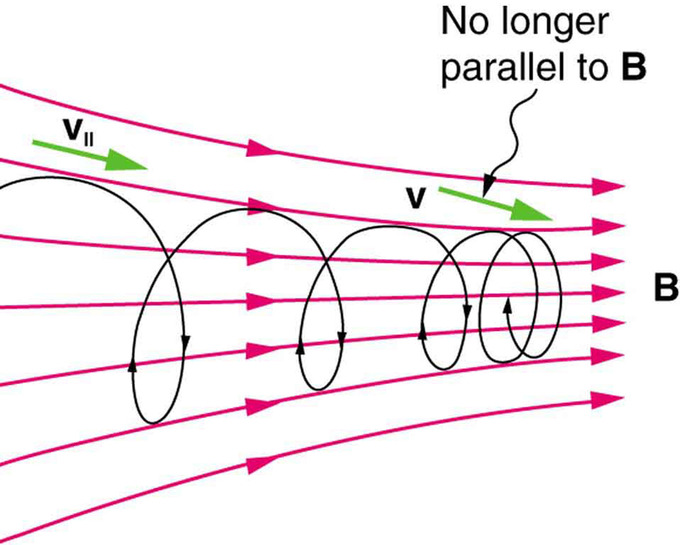
Helical Motion and Magnetic Mirrors : When a charged particle moves along a magnetic field line into a region where the field becomes stronger, the particle experiences a force that reduces the component of velocity parallel to the field. This force slows the motion along the field line and here reverses it, forming a “magnetic mirror. “
The motion of charged particles in magnetic fields are related to such different things as the Aurora Borealis or Aurora Australis (northern and southern lights) and particle accelerators. Charged particles approaching magnetic field lines may get trapped in spiral orbits about the lines rather than crossing them , as seen above. Some cosmic rays, for example, follow the Earth’s magnetic field lines, entering the atmosphere near the magnetic poles and causing the southern or northern lights through their ionization of molecules in the atmosphere. Those particles that approach middle latitudes must cross magnetic field lines, and many are prevented from penetrating the atmosphere. Cosmic rays are a component of background radiation; consequently, they give a higher radiation dose at the poles than at the equator.
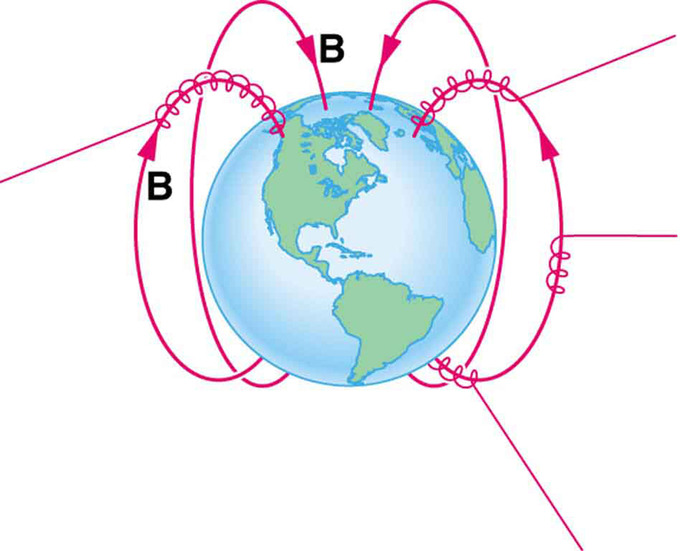
Charged Particles Spiral Along Earth’s Magnetic Field Lines : Energetic electrons and protons, components of cosmic rays, from the Sun and deep outer space often follow the Earth’s magnetic field lines rather than cross them. (Recall that the Earth’s north magnetic pole is really a south pole in terms of a bar magnet. )
Examples and Applications
Cyclotrons, magnetrons, and mass spectrometers represent practical technological applications of electromagnetic fields.
- Discuss application of mass spectrometers, movement of charged particles in a cyclotron, and how microwaves are generated in the cavity magnetron
Examples and Applications – Motion of a Charged Particle in a Magnetic Field
Recall that the charged particles in a magnetic field will follow a circular or spiral path depending on the alignment of their velocity vector with the magnetic field vector. The consequences of such motion can have profoundly practical applications. Many technologies are based on the motion of charged particles in electromagnetic fields. We will explore some of these, including the cyclotron and synchrotron, cavity magnetron, and mass spectrometer.
Cyclotrons and Synchrotrons
A cyclotron is a type of particle accelerator in which charged particles accelerate outwards from the center along a spiral path. The particles are held to a spiral trajectory by a static magnetic field and accelerated by a rapidly varying (radio frequency ) electric field.
Cyclotron Sketch : Sketch of a particle being accelerated in a cyclotron, and being ejected through a beamline.
Cyclotrons accelerate charged particle beams using a high frequency alternating voltage which is applied between two “D”-shaped electrodes (also called “dees”). An additional static magnetic field is applied in perpendicular direction to the electrode plane, enabling particles to re-encounter the accelerating voltage many times at the same phase. To achieve this, the voltage frequency must match the particle’s cyclotron resonance frequency,
\[\mathrm { f } = \dfrac { \mathrm { qB } } { 2 \pi \mathrm { m } }\]
with the relativistic mass m and its charge q . This frequency is given by equality of centripetal force and magnetic Lorentz force. The particles, injected near the center of the magnetic field, increase their kinetic energy only when recirculating through the gap between the electrodes; thus they travel outwards along a spiral path. Their radius will increase until the particles hit a target at the perimeter of the vacuum chamber, or leave the cyclotron using a beam tube, enabling their use. The particles accelerated by the cyclotron can be used in particle therapy to treat some types of cancer. Additionally, cyclotrons are a good source of high-energy beams for nuclear physics experiments.
A synchrotron is an improvement upon the cyclotron in which the guiding magnetic field (bending the particles into a closed path) is time-dependent, being synchronized to a particle beam of increasing kinetic energy. The synchrotron is one of the first accelerator concepts that enable the construction of large-scale facilities, since bending, beam focusing and acceleration can be separated into different components.
Cavity Magnetron
The cavity magnetron is a high-powered vacuum tube that generates microwaves using the interaction of a stream of electrons with a magnetic field. All cavity magnetrons consist of a hot cathode with a high (continuous or pulsed) negative potential created by a high-voltage, direct-current power supply. The cathode is built into the center of an evacuated, lobed, circular chamber. A magnetic field parallel to the filament is imposed by a permanent magnet. The magnetic field causes the electrons, attracted to the (relatively) positive outer part of the chamber, to spiral outward in a circular path, a consequence of the Lorentz force. Spaced around the rim of the chamber are cylindrical cavities. The cavities are open along their length and connect the common cavity space. As electrons sweep past these openings, they induce a resonant, high-frequency radio field in the cavity, which in turn causes the electrons to bunch into groups.
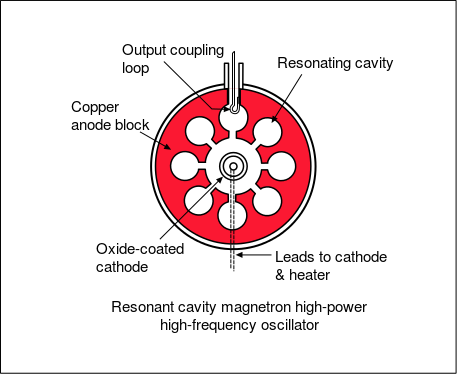
Cavity Magnetron Diagram : A cross-sectional diagram of a resonant cavity magnetron. Magnetic lines of force are parallel to the geometric axis of this structure.
The sizes of the cavities determine the resonant frequency, and thereby the frequency of emitted microwaves. The magnetron is a self-oscillating device requiring no external elements other than a power supply. The magnetron has practical applications in radar, heating (as the primary component of a microwave oven), and lighting.
Mass Spectrometry
Mass spectrometry is an analytical technique that measures the mass-to-charge ratio of charged particles. It is used for determining masses of particles and determining the elemental composition of a sample or molecule.
Mass analyzers separate the ions according to their mass-to-charge ratio. The following two laws govern the dynamics of charged particles in electric and magnetic fields in a vacuum:
\[\mathrm { F } = \mathrm { Q } ( \mathrm { E } + \mathrm { v } \times \mathrm { B } ) \text{ (Lorentz force)}\]
\[\mathrm { F } = \mathrm { ma }\]
Equating the above expressions for the force applied to the ion yields:
\[( \mathrm { m } / \mathrm { Q } ) \mathrm { a } = \mathrm { E } + \mathrm { v } \times \mathrm { B }\]
This differential equation along with initial conditions completely determines the motion of a charged particle in terms of m/Q. There are many types of mass analyzers, using either static or dynamic fields, and magnetic or electric fields, but all operate according to the above differential equation.
The following figure illustrates one type of mass spectrometer. The deflections of the particles are dependent on the mass-to-charge ratio. In the case of isotopic carbon dioxide, each molecule has the same charge, but different masses. The mass spectrometer will segregate the particles spatially allowing a detector to measure the mass-to-charge ratio of each particle. Since the charge is known, the absolute mass can be determined trivially. The relative abundances can be inferred from counting the number of particles of each given mass.
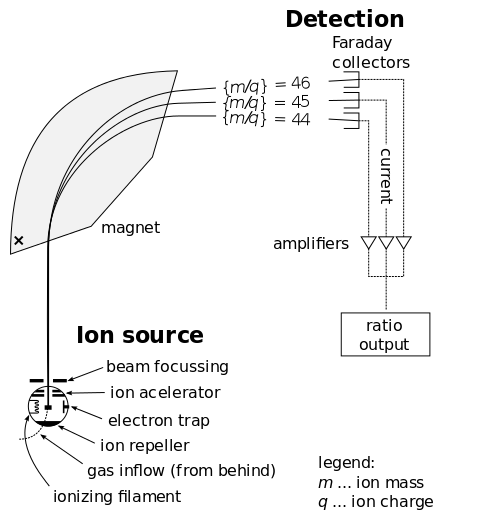
Mass Spectrometry : Schematics of a simple mass spectrometer with sector type mass analyzer. This one is for the measurement of carbon dioxide isotope ratios (IRMS) as in the carbon-13 urea breath test.
- The force on a charged particle due to an electric field is directed parallel to the electric field vector in the case of a positive charge, and anti-parallel in the case of a negative charge. It does not depend on the velocity of the particle.
- In contrast, the magnetic force on a charge particle is orthogonal to the magnetic field vector, and depends on the velocity of the particle. The right hand rule can be used to determine the direction of the force.
- An electric field may do work on a charged particle, while a magnetic field does no work.
- The Lorentz force is the combination of the electric and magnetic force, which are often considered together for practical applications.
- Electric field lines are generated on positive charges and terminate on negative ones. The field lines of an isolated charge are directly radially outward. The electric field is tangent to these lines.
- Magnetic field lines, in the case of a magnet, are generated at the north pole and terminate on a south pole. Magnetic poles do not exist in isolation. Like in the case of electric field lines, the magnetic field is tangent to the field lines. Charged particles will spiral around these field lines.
- Newton’s first law of motion states that if an object experiences no net force, then its velocity is constant.
- A particle with constant velocity will move along a straight line through space.
- If a charged particle’s velocity is completely parallel to the magnetic field, the magnetic field will exert no force on the particle and thus the velocity will remain constant.
- In the case that the velocity vector is neither parallel nor perpendicular to the magnetic field, the component of the velocity parallel to the field will remain constant.
- The magnetic field does no work, so the kinetic energy and speed of a charged particle in a magnetic field remain constant.
- The magnetic force, acting perpendicular to the velocity of the particle, will cause circular motion.
- The centripetal force of the particle is provided by magnetic Lorentzian force so that \(\mathrm { qvB } = \frac { \mathrm { mv } ^ { 2 } } { \mathrm { r } }\).
- Solving for r above yields the gryoradius, or the radius of curvature of the path of a particle with charge q and mass m moving in a magnetic field of strength B. The gryoradius is then given by \(\mathrm{ \Gamma = \frac { m v } { q B }}\).
- The cyclotron frequency (or, equivalently, gyrofrequency) is the number of cycles a particle completes around its circular circuit every second and is given by \(\mathrm { f } = \frac { \mathrm { q } \mathrm { B } } { 2 \pi \mathrm { m } }\).
- Previously, we have seen that circular motion results when the velocity of a charged particle is perpendicular to the magnetic field. The speed and kinetic energy of the particle remain constant, but the direction is altered at each instant by the perpendicular magnetic force.
- If the velocity is not perpendicular to the magnetic field, we consider only the component of v that is perpendicular to the field when making our calculations.
- The component of the velocity parallel to the field is unaffected, since the magnetic force is zero for motion parallel to the field. This produces helical motion.
- Charges may spiral along field lines. If the strength of the magnetic field increases in the direction of motion, the field will exert a force to slow the charges and even reverse their direction. This is known as a magnetic mirror.
- A cyclotron is a type of particle accelerator in which charged particles accelerate outwards from the center along a spiral path. The particles are held to a spiral trajectory by a static magnetic field and accelerated by a rapidly varying electric field.
- The cavity magnetron is a high-powered vacuum tube that generates microwaves using the interaction of a stream of electrons with a magnetic field. The magnetron has applications in radar, heating, and lighting.
- Mass spectrometers measure the mass-to-charge ratio of charged particles through the use of electromagnetic fields to segregate particles with different masses and/or charges. It can be used to determine the elemental composition of a molecule or sample.
- orthogonal : Of two objects, at right angles; perpendicular to each other.
- straight-line motion : motion that proceeds in a single direction.
- gyroradius : The radius of the circular motion of a charged particle in the presence of a uniform magnetic field.
- cyclotron frequency : The frequency of a charged particle moving perpendicular to the direction of a uniform magnetic field B (constant magnitude and direction). Given by the equality of the centripetal force and magnetic Lorentz force.
- helical motion : The motion that is produced when one component of the velocity is constant in magnitude and direction (i.e., straight-line motion) while the other component is constant in speed but uniformly varies in direction (i.e., circular motion). It is the superposition of straight-line and circular motion.
- magnetic mirror : A magnetic field configuration where the field strength changes when moving along a field line. The mirror effect results in a tendency for charged particles to bounce back from the high field region.
- cyclotron : An early particle accelerator in which charged particles were generated at a central source and accelerated spirally outward through a fixed magnetic and alternating electric fields.
- mass spectrometer : A device used in mass spectrometry to discover the mass composition of a given substance.
- magnetron : A device in which electrons are made to resonate in a specially shaped chamber and thus produce microwave radiation; used in radar, and in microwave ovens.
LICENSES AND ATTRIBUTIONS
CC LICENSED CONTENT, SHARED PREVIOUSLY
- Curation and Revision. Provided by : Boundless.com. License : CC BY-SA: Attribution-ShareAlike
CC LICENSED CONTENT, SPECIFIC ATTRIBUTION
- OpenStax College, College Physics. September 18, 2013. Provided by : OpenStax CNX. Located at : http://cnx.org/content/m42312/latest/?collection=col11406/1.7 . License : CC BY: Attribution
- OpenStax College, College Physics. September 18, 2013. Provided by : OpenStax CNX. Located at : http://cnx.org/content/m42372/latest/?collection=col11406/1.7 . License : CC BY: Attribution
- OpenStax College, College Physics. September 18, 2013. Provided by : OpenStax CNX. Located at : http://cnx.org/content/m42375/latest/?collection=col11406/1.7 . License : CC BY: Attribution
- OpenStax College, College Physics. September 18, 2013. Provided by : OpenStax CNX. Located at : http://cnx.org/content/m42308/latest/?collection=col11406/1.7 . License : CC BY: Attribution
- Electric force. Provided by : Wikipedia. Located at : en.Wikipedia.org/wiki/Electric_force . License : CC BY-SA: Attribution-ShareAlike
- Electric field. Provided by : Wikipedia. Located at : en.Wikipedia.org/wiki/Electric_field . License : CC BY-SA: Attribution-ShareAlike
- OpenStax College, College Physics. September 18, 2013. Provided by : OpenStax CNX. Located at : http://cnx.org/content/m42310/latest/?collection=col11406/1.7 . License : CC BY: Attribution
- Magnetic field lines. Provided by : Wikipedia. Located at : en.Wikipedia.org/wiki/Magnetic_field_lines%23Magnetic_field_lines . License : CC BY-SA: Attribution-ShareAlike
- OpenStax College, College Physics. September 18, 2013. Provided by : OpenStax CNX. Located at : http://cnx.org/content/m42370/latest/?collection=col11406/1.7 . License : CC BY: Attribution
- Magnetic force. Provided by : Wikipedia. Located at : en.Wikipedia.org/wiki/Magnetic_force%23History . License : CC BY-SA: Attribution-ShareAlike
- orthogonal. Provided by : Wiktionary. Located at : en.wiktionary.org/wiki/orthogonal . License : CC BY-SA: Attribution-ShareAlike
- OpenStax College, College Physics. December 10, 2012. Provided by : OpenStax CNX. Located at : http://cnx.org/content/m42312/latest/?collection=col11406/1.7 . License : CC BY: Attribution
- Magnetic field lines. Provided by : Wikipedia. Located at : en.Wikipedia.org/wiki/Magnetic_field_lines%23Magnetic_field_lines . License : Public Domain: No Known Copyright
- OpenStax College, College Physics. November 14, 2012. Provided by : OpenStax CNX. Located at : http://cnx.org/content/m42372/latest/?collection=col11406/1.7 . License : CC BY: Attribution
- OpenStax College, College Physics. September 17, 2013. Provided by : OpenStax CNX. Located at : http://cnx.org/content/m42372/latest/?collection=col11406/1.7 . License : CC BY: Attribution
- Newton's laws. Provided by : Wikipedia. Located at : en.Wikipedia.org/wiki/Newton's_laws . License : CC BY-SA: Attribution-ShareAlike
- Boundless. Provided by : Boundless Learning. Located at : www.boundless.com//physics/definition/straight-line-motion . License : CC BY-SA: Attribution-ShareAlike
- OpenStax College, College Physics. November 28, 2012. Provided by : OpenStax CNX. Located at : http://cnx.org/content/m42372/latest/?collection=col11406/1.7 . License : CC BY: Attribution
- Cyclotron resonance. Provided by : Wikipedia. Located at : en.Wikipedia.org/wiki/Cyclotron_resonance . License : CC BY-SA: Attribution-ShareAlike
- Cyclotron. Provided by : Wikipedia. Located at : en.Wikipedia.org/wiki/Cyclotron . License : CC BY-SA: Attribution-ShareAlike
- Gyroradius. Provided by : Wikipedia. Located at : en.Wikipedia.org/wiki/Gyroradius . License : CC BY-SA: Attribution-ShareAlike
- OpenStax College, College Physics. September 17, 2013. Provided by : OpenStax CNX. Located at : http://cnx.org/content/m42375/latest/?collection=col11406/1.7 . License : CC BY: Attribution
- gyroradius. Provided by : Wiktionary. Located at : en.wiktionary.org/wiki/gyroradius . License : CC BY-SA: Attribution-ShareAlike
- cyclotron frequency. Provided by : Wikipedia. Located at : en.Wikipedia.org/wiki/cyclotron%20frequency . License : CC BY-SA: Attribution-ShareAlike
- OpenStax College, College Physics. January 16, 2015. Provided by : OpenStax CNX. Located at : http://cnx.org/content/m42375/latest/?collection=col11406/1.7 . License : CC BY: Attribution
- OpenStax College, College Physics. November 26, 2012. Provided by : OpenStax CNX. Located at : http://cnx.org/content/m42375/latest/?collection=col11406/1.7 . License : CC BY: Attribution
- Cyclotron. Provided by : Wikipedia. Located at : en.Wikipedia.org/wiki/Cyclotron . License : Public Domain: No Known Copyright
- Magnetic mirror. Provided by : Wikipedia. Located at : en.Wikipedia.org/wiki/Magnetic_mirror . License : CC BY-SA: Attribution-ShareAlike
- Boundless. Provided by : Boundless Learning. Located at : www.boundless.com//physics/definition/helical-motion . License : CC BY-SA: Attribution-ShareAlike
- magnetic mirror. Provided by : Wikipedia. Located at : en.Wikipedia.org/wiki/magnetic%20mirror . License : CC BY-SA: Attribution-ShareAlike
- OpenStax College, College Physics. November 27, 2012. Provided by : OpenStax CNX. Located at : http://cnx.org/content/m42375/latest/?collection=col11406/1.7 . License : CC BY: Attribution
- Mass spectrometers. Provided by : Wikipedia. Located at : en.Wikipedia.org/wiki/Mass_spectrometers . License : CC BY-SA: Attribution-ShareAlike
- Magnetron. Provided by : Wikipedia. Located at : en.Wikipedia.org/wiki/Magnetron . License : CC BY-SA: Attribution-ShareAlike
- Synchrotron. Provided by : Wikipedia. Located at : en.Wikipedia.org/wiki/Synchrotron . License : CC BY-SA: Attribution-ShareAlike
- Cyclotron. Provided by : Wikipedia. Located at : http://en.Wikipedia.org/wiki/Cyclotron . License : CC BY-SA: Attribution-ShareAlike
- cyclotron. Provided by : Wiktionary. Located at : en.wiktionary.org/wiki/cyclotron . License : CC BY-SA: Attribution-ShareAlike
- magnetron. Provided by : Wiktionary. Located at : en.wiktionary.org/wiki/magnetron . License : CC BY-SA: Attribution-ShareAlike
- mass spectrometer. Provided by : Wiktionary. Located at : en.wiktionary.org/wiki/mass_spectrometer . License : CC BY-SA: Attribution-ShareAlike
- Cyclotron. Provided by : Wikipedia. Located at : http://en.Wikipedia.org/wiki/Cyclotron . License : Public Domain: No Known Copyright
- Mass spectrometers. Provided by : Wikipedia. Located at : en.Wikipedia.org/wiki/Mass_spectrometers . License : Public Domain: No Known Copyright
- Magnetron. Provided by : Wikipedia. Located at : en.Wikipedia.org/wiki/Magnetron . License : Public Domain: No Known Copyright

- [ 24 Jul 2023 ] AERODIUM Mirabel Experience (Video) Promos
- [ 26 May 2023 ] Unveiling an Exhilarating Indoor Wind Tunnel in Kyiv, Ukraine Articles
- [ 3 May 2023 ] Top 5 Most Viewed Indoor Skydiving Videos of 2022 Articles
- [ 2 May 2023 ] Máté Feith’s Golden Freestyle at The 2023 World Indoor Skydiving Championships (Video) Competitions
- [ 2 May 2023 ] Máté Feith’s Routine at Luxfly Grand Opening 2021 (Video) Promos
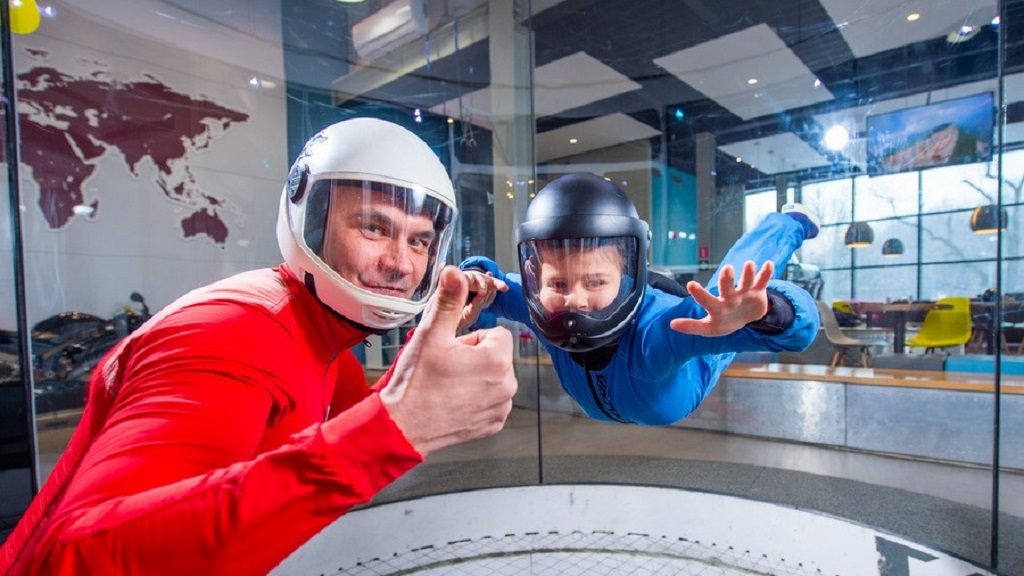
Have you ever dreamed of flying like a superhero ? Now you can! From children to seniors everybody can fly in a Wind Tunnel. Make your first flight and try this incredible new experience or discover bodyflight as an exciting new sport .
Wind Tunnel Info
IndoorSkydiving.World is a separate activity from Vacuum . If you wish to suggest an edit or submit a new tunnel please contact us .
Vacuum Indoor Skydiving Moscow is where the dream of human flight becomes a reality. From children to seniors everybody can fly , just for fun or to start bodyflight as a new exciting sport .
CONTACTS & BOOKING
Wind tunnel and hotels map, first flight & groups.

You’ll fall in love with the sensation and the freedom that comes with floating on air . Millions of people worldwide have experienced the power of flight in a safe environment . Are you ready to fly?
Virtually anyone from children to seniors can fly. For restrictions and more info read the FAQs or contact the Wind Tunnel.
Yes, the facility and gears are designed for your safety. And highly trained flight instructors will be with you the entire time.
From birthday parties to corporate events and everything in between, a wind tunnel is the perfect venue for groups of all sizes with everything you need for an over-the-top experience.
SPORT FLYERS & TEAMS
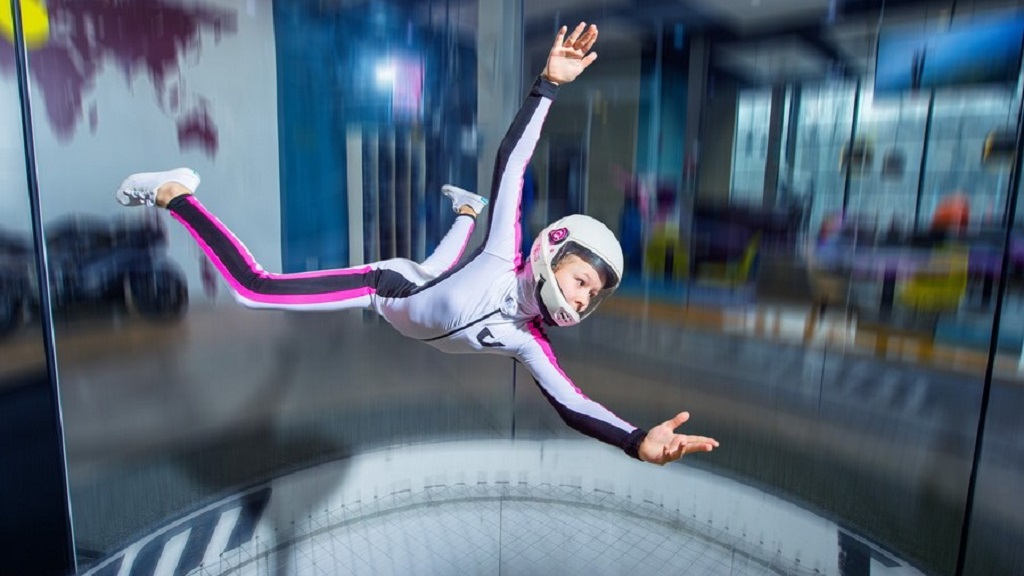
The Sport of Indoor Skydiving , also known as Bodyflight , is one of the most exciting and fastest growing sports in the world. Nearly anyone can become a skilled flyer through a progression system and personalized coaching.
For bulk time , off-peak rates and special offers contact the wind tunnel . You can also see which are the lowest rates in Russia and worldwide here .
See below Coaches and Events at Vacuum or look for more wind tunnels in Russia or around the world .
COACHES & COMPETITORS
Contact Vacuum for more options or submit a new coach .
EVENTS & COMPETITIONS
Submit an event or find events in Russia and worldwide .
TECHNICAL INFORMATION

TRAVELS & ATTRACTIONS
Discover Moscow , its attractions and the surrounding area with our best deals .
CAR HIRE DEALS NEARBY Vacuum
More wind tunnels, give your feedback, stay in the loop, you may like.
(c) 2010 IndoorSkydiving.World

IMAGES
VIDEO
COMMENTS
1. Decay of a neutron happens when it is energetically possible, i.e. the decay fragments are more bound than the nucleus that decays via neutron decay. (beta-). There is no such case for the alpha and that is why it is stable. Proton decay depending on the new theory, would happen through exchanges of new, non standard model, particles within ...
Alpha particles, also called alpha rays or alpha radiation, consist of two protons and two neutrons bound together into a particle identical to a helium-4 nucleus. They are generally produced in the process of alpha decay but may also be produced in other ways. Alpha particles are named after the first letter in the Greek alphabet, α.The symbol for the alpha particle is α or α 2+.
Po-210 has two possible alpha particle emissions, the most probable being 5304 keV (100%), and the mean distance travelled in air will be about 3.5 cm. Some obscure or more exotic radioactive materials have much higher alpha particle emission energy. For example, Po-212 has an alpha particle emission of over 10 MeV with a mean range in air of ...
Consequently, the penetration depth of alpha particles is very small compared to the other radiations. For low density materials, the range [2] of 5.5 MeV alphas (from Am-241) is between 4.5 to 5 mg/cm 2; higher density materials give a range between 5 and 12 mg/cm 2. The table below gives some specific values.
Three quantities may be directly measured: (a) the energy of the alpha particle after it has passed through a known amount of gas, (b) the spread in its energy, and (c) the total ... Before using the compressed gas cylinders to add gas to the vacuum chamber check with the TA for procedure. When you finish the experiment and leave be sure that ...
alpha particle, positively charged particle, identical to the nucleus of the helium-4 atom, spontaneously emitted by some radioactive substances, consisting of two protons and two neutrons bound together, thus having a mass of four units and a positive charge of two. Discovered and named (1899) by Ernest Rutherford, alpha particles were used by him and coworkers in experiments to probe the ...
plot of the energy loss of the ionizing radiation as the particles travel through matter is called a Bragg plot. The Bragg plot will show a peak just before the particles come to rest. Fig.2 shows a Bragg plot for 5.49 MeV Alpha par-ticles in air at STP. The peak in this case corresponds to an Alpha particle energy of about 1 MeV. 3
An alpha particle is a particle consisting of two protons, two neutrons, and no electrons. Essentially, it is a helium-4 nucleus. Other names for alpha particles are alpha rays or alpha radiation. The symbol for an alpha particle is α, α 2+, He 2+, or 42 He 2+. Usually, alpha particles result from alpha radioactive decay, but they form via ...
A single particle's mass is 4 amu (6.642×10−4 g), according to Britannica Alpha particles are emitted from heavy radioactive elements (both naturally occurring and man-made), including uranium ...
Alpha particles consist of two protons and two neutrons bound together into a particle identical to a helium nucleus. Alpha particles are relatively large and carry a double positive charge. They are not very penetrating, and a piece of paper can stop them. They travel only a few centimeters but deposit all their energies along their short paths.
The α-particle detection has a pivotal role in radiation measurement. Standoff α-particle detection has advantages such as high efficiency and safety compared with the traditional method. However, the low yield of the characteristic signal significantly limits the broad application of this technique.
The alpha particle blobs further getting smaller with increasing distance. Approximately at 4 cm, the alpha particles are much smaller (Fig. 4) and if we further increase the distance to 4.5 cm, they disappear. Figure 4. The alpha particles losing most of their energy and almost diminishing at a distance of 4 cm.
The particles travel at 5 to 7 % of the speed of light or 20,000,000 metres per second and has a mass approximately equivalent to 4 protons. ... Alpha-particle radiation is normally only a safety concern if the radioactive decay occurs from an atom that is already inside the body or a cell. Alpha-particle emitters are particularly dangerous if ...
The blue glow of Cherenkov radiation, seen here at a nuclear-reactor core, can also be generated by subatomic particles speeding through a vacuum. Credit: US Department of Energy/SPL. Thanks to a ...
1. Vacuum Chamber. The vacuum chamber is made of pyrex glass and is mounted inside a dark wooden box. The Silicon detector must be pro-tected from room light since photons cause a noise background which spoils the resolution of the detector. Do not open the glassware. The alpha source could, over a long time, shed some radioactive dust. The
The energy of alpha particles varies, with higher energy alpha particles being emitted from larger nuclei, but most alpha particles have energies of between 3 and 7 MeV, corresponding to extremely long and extremely short half-lives of alpha-emitting nuclides, respectively.. This energy is a substantial amount of energy for a single particle, but their high mass means alpha particles have a ...
The video talks about the particle-wave duality of an electron. So a single electron can act as a wave, but when it hits something it hits only one place. Is that the same for photons? Thought Experiment: Out in the vacuum of space there is a huge hollow sphere 1 light year in radius. Inside is just vaccuum, and at the exact center a flash of ...
3. How do alpha particles behave in a vacuum? Alpha particles in a vacuum travel in straight lines at high speeds due to their mass and charge. 4. What can alpha particles do in a vacuum? In a vacuum, alpha particles can penetrate materials, cause ionization, and produce visible light when they interact with gas molecules. 5.
Alpha-Particle Spectroscopy and Ranges in Air. Abstract: The present study investigated energies of alpha-particles emitted by the triple-alpha sources (239Pu, 241Am and 244Cm) in a vacuum and ...
The present study investigated energies of alpha-particles emitted by the triple-alpha sources (239 Pu, 241 Am and 244 Cm) in a vacuum and measure the alpha particle properties at different pressures.
A further difference between magnetic and electric forces is that magnetic fields do not net work, since the particle motion is circular and therefore ends up in the same place. We express this mathematically as: W = ∮B ⋅ dr = 0 (21.4.5) (21.4.5) W = ∮ B ⋅ d r = 0.
Abstract. The use of superparamagnetic iron oxide nanoparticles (SPIONs) and radiolabelled nanoparticles (NPs) has grown considerably over the recent years, and the SPIONs labelled with medicinal radionuclides offer new opportunities in multimodal diagnostics and in the drug-delivery systems for targeted alpha-particle therapy (TAT) driven by magnetic field gradient or by biologically active ...
Vacuum Indoor Skydiving Moscow is where the dream of human flight becomes a reality. From children to seniors everybody can fly, just for fun or to start bodyflight as a new exciting sport. ... FIRST FLIGHT & GROUPS SPORT FLYERS & EVENTS TECHNICAL INFORMATION TRAVEL INFORMATION NOTICE. IndoorSkydiving.World is a separate activity from Vacuum.